Cowpea: A nutrient-rich pulse for extreme environments
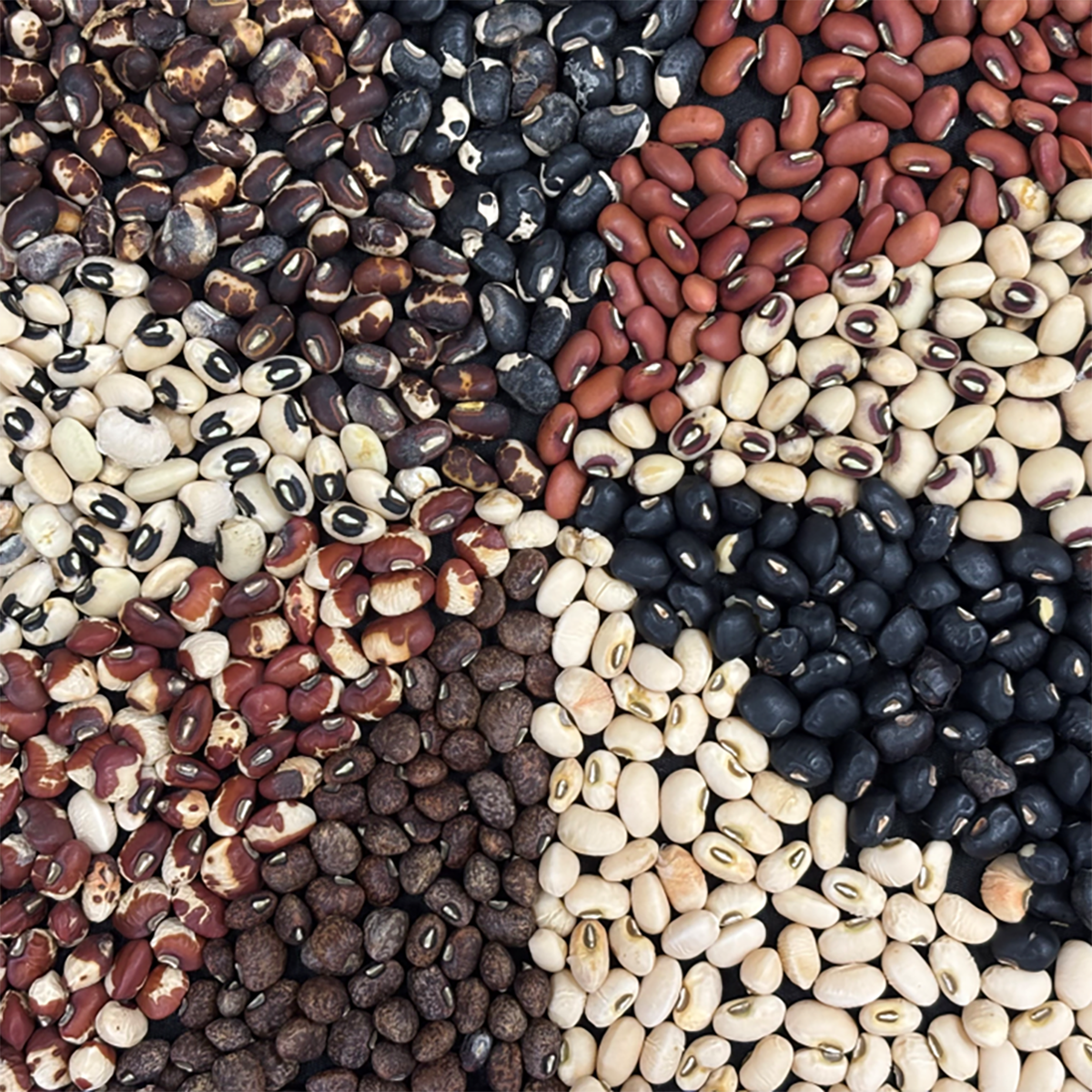
Cowpea is a highly adaptable and nutritious legume known for its drought tolerance, nitrogen-fixing ability, and ecological benefits, making it valuable for sustainable agriculture. Its genetic diversity and early maturity enable it to thrive in diverse environments, including arid regions and U.S. farmlands, with growing interest in its revival due to food security concerns. Ongoing research is uncovering key physiological and genetic traits that enhance cowpea’s resilience to drought and heat stress, particularly during critical growth stages, to support breeding efforts for future climate challenges.
Cowpea [Vigna unguiculata (L.) Walp] is gaining recognition as a "miracle crop" thanks to its significant ecological contributions and health benefits. This warm-season legume, known as southern pea or black-eyed pea, shows substantial genetic diversity in plant architecture, ranging from short and bushy to tall and vine-like with growth forms that can be erect, semi-erect, prostrate, or climbing (Sheahan, 2012). Cowpea is a diploid species (2n=22 chromosomes) with a genome size of about 641 million base pairs and is estimated to have about 44,000 genes. It demonstrates a broad gene pool and adaptability (Lonardi et al., 2019). Depending on genotype selection and environmental factors, its maturity period can vary from 50 to 160 days. This characteristic of early maturation has earned cowpea the label of a “hungry-season crop.”. The plant’s versatility is further exemplified by its varied uses: young leaves and immature pods are consumed as vegetables, mature seeds are harnessed as pulses, and leaves and branches serve as fodder for livestock (Owade et al., 2020).
Cowpea has a rich history of global dissemination, facilitated by trade routes that allowed it to flourish as a vital food and forage crop in regions across Africa, Asia, and the Americas. In the United States, cowpeas were introduced in the 1700s, primarily as a fodder crop, especially in southern states. Over the years, it transitioned into a significant grain crop, with notable cultivation from the 1930s to the 1960s, when more than 250,000 hectares were dedicated to production, culminating in a yield of nearly 33,503 metric tons in 1961. However, the rise of soybeans as a dominant crop led to a decline in cowpea cultivation. As regenerative agriculture becomes increasingly important in the face of production and food security challenges, the remarkable qualities of cowpea are being re-evaluated, potentially paving the way for a resurgence of this resilient crop in global agriculture. Cowpea is experiencing a revival with about 12,927 metric tons of dry seeds produced in 2023 across 7280 hectares, mainly in Georgia, Texas, Arkansas, and California (FAOSTAT, 2023).
Cowpea is a robust legume that thrives in arid conditions. It demonstrates remarkable drought tolerance (Turk et al., 1980). However, despite its drought hardiness, not all cowpea genotypes perform equally under drought conditions as noted in millet (Bista & Bheemanahalli, 2024). While some high-yielding cowpea genotypes that thrive in irrigated environments are susceptible to drought, few exhibit drought tolerance (Singini et al., 2024). This variation highlights the need to understand and harness key physiological traits contributing to drought resilience. One such trait is cowpea’s ability to maintain a relatively stable leaf water status during water deficit (Nunes et al., 2022), which is supported by a diverse array of adaptive responses, ranging from cellular mechanisms to whole-plant strategies.
Cowpea offers greater genetic diversity and resilience than other crops, making it a smart alternative for extremely challenging growing conditions. Moreover, there is potential for expanding cowpea cultivation in the U.S. through double cropping practices in areas where wheat, corn, and sorghum are grown, particularly by employing cowpea varieties that reach maturity in approximately 60 days.
A multipurpose crop
Cowpea is a highly adaptable crop with significant agronomic, nutritional, and ecological benefits. Its ability to fix nitrogen, improve soil health, suppress weeds, provide valuable forage, and attract beneficial insects makes it a sustainable and valuable component of diverse agricultural systems. Cowpea forms a symbiotic relationship with rhizobia bacteria, allowing it to fix atmospheric nitrogen into a usable form for the plant (Awonaike et al., 1990) reducing the need for chemical fertilizer. The green fodder, including the vines and leaves, can be harvested and dried as hay or ensiled as silage, providing high-quality forage for livestock such as cattle, sheep, and goats. Additionally, cowpea is an effective cover crop, improving soil structure, water infiltration, and water-holding capacity.
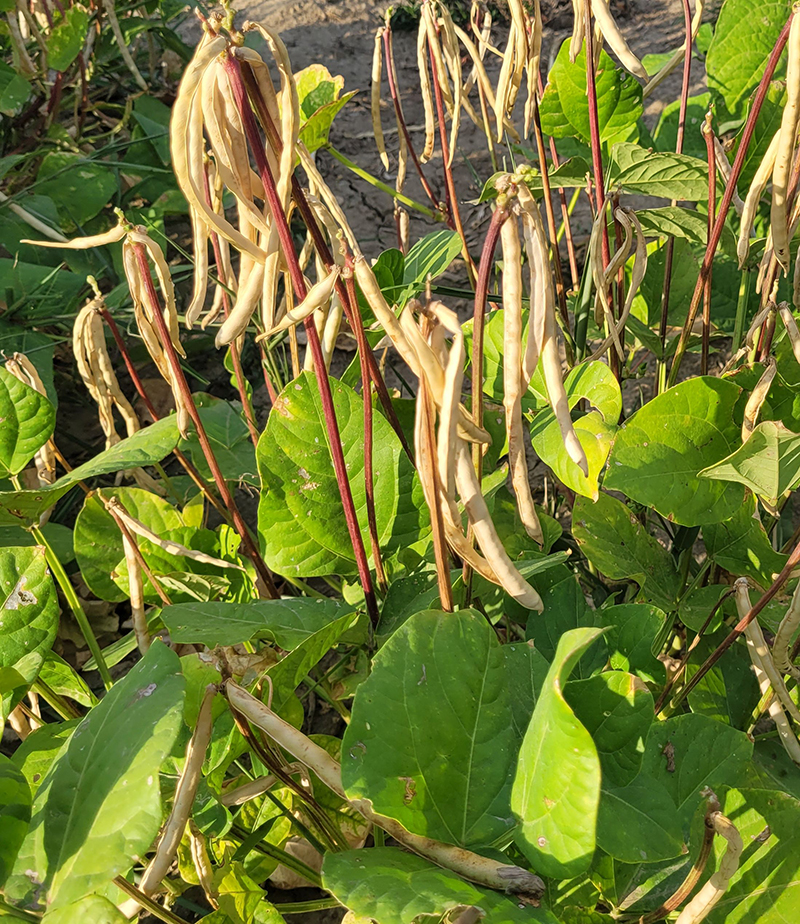
Cowpea is a nutritious legume that provides a balanced source of carbohydrates and protein essential for nutritional security. It contains about 61.8 g of carbohydrates, 23.8 g of protein, and 2.07 g of fat per 100 grams of seeds (USDA, 2019). Cowpeas' high dietary fiber content (34 g, of which 29.8 g is insoluble fiber) supports blood glucose control and gut health (Mallillin et al., 2008). Cowpeas are primarily composed of starch and contain a lower lipid profile, in which approximately 70.7% of the total fat is unsaturated, contributing to heart health. Additionally, resistant starch and amylose aid digestion by slowing glucose release into the bloodstream, which helps decrease glucose absorption in the intestines (Abebe & Alemayehu, 2022). Compared with other starch sources, cowpeas offer a glycemic index, thus lower digestibility, which can help reduce insulin responses and hunger (Ma et al., 2017). In addition, they are also rich in essential nutrients like phosphorus, potassium, zinc, and vitamins A and C and folate (Affrifah et al., 2022). Cowpea also contains beneficial phytochemicals, including phenolic compounds and flavonoids, that support various health benefits, such as anti-inflammatory and anti-diabetic properties (Jayathilake et al., 2018). Medicinally, the leaves and pods are used for ailments like measles while the seeds have diuretic properties. Additionally, cowpeas are culturally significant and are thought to bring good luck and prosperity when consumed on New Year’s Eve.
Resilience to drought
Cowpeas adopt various strategies to survive and reproduce in response to drought, including escape, where they accelerate flowering and complete their life cycle to reduce drought exposure (Fatokun et al., 2012). They also employ water-saving strategies like stomatal closure, reduced leaf area, and wax accumulation (Singh & Reddy, 2011). Additionally, some genotypes adapt a water-spending approach by adjusting root architecture to access deeper soil moisture, mitigating drought effects (Matsui & Singh, 2003). Drought tolerance mechanisms involve plant adaptations at the cellular level to cope with water scarcity, such as accumulating compatible solutes (like proline and sugars) and producing antioxidants to maintain cell turgor by scavenging reactive oxygen species (Goufo et al., 2017; Seki et al., 2007).
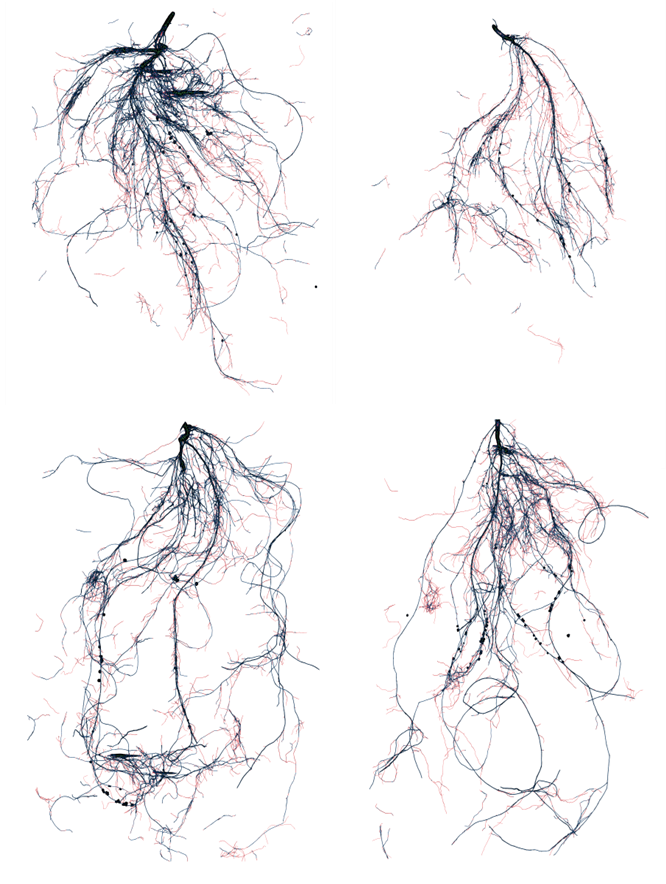
Our lab (Plant Stress Physiology Laboratory) at Mississippi State University has been conducting interdisciplinary research to discover traits that can improve cowpea's adaptability to drought. We hypothesize that the growth and productivity of crops under drought are influenced by physiological mechanisms that regulate tissue–water dynamics and carbon assimilation during stress and recovery. A graduate student in the lab, Mr. Sujan Poudel, has sought to understand how biomass is sustained by key traits associated with drought avoidance, such as the root systems' ability to access water (water mining) and the regulation of stomatal conductance to minimize water loss (water saving). Under low soil moisture, roots sense drought and produce abscisic acid hormone, causing stomatal closure to minimize water loss. Shallow-rooted cowpea plants were more sensitive to drought (Poudel et al., 2025), resulting in reduced assimilation or biomass compared with robust-rooted genotypes (Figure 1). Further, multi-stage drought-resilience experiments revealed that subjecting cowpea to stress during the nodule initiation had adverse effects on physiological functions and nodal branching compared with the reproductive stage. Interestingly, gas exchange and vegetative tissues showed strong recovery at the vegetative stage. Meanwhile, drought during peak blooming induced irreversible damage to key traits (flower abscission and abortion) that determine yield potential. Based on seed number and weight, the order of cowpea growth stage resilience to drought followed the flowering (R1) < seed fill (R4) < nodulation initiation (V2) < branching (V4) (Figure 2).
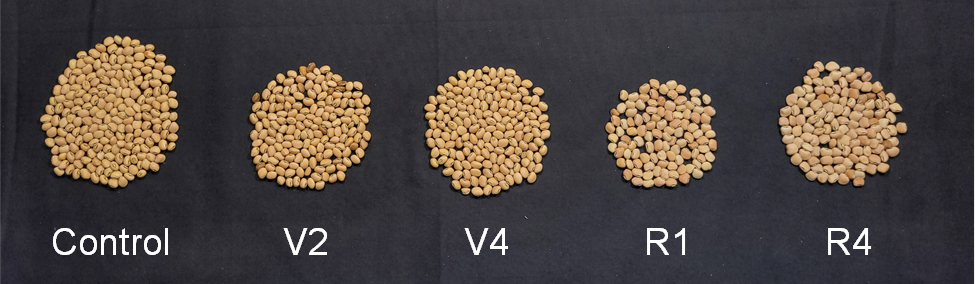
The yield-attributing traits among cowpea genotypes during the different growth stages of drought stress suggest a significant potential for stage-specific breeding, selection, and management strategies. Notably, the early bloom stage requires the most water due to reproductive energy demands, making it the most sensitive stage. Genotypes with better root systems at the vegetative stage showed great drought resilience at the reproductive stage and maintained higher yields, suggesting a robust causal correlation between roots and resilience throughout the cowpea life cycle. To identify the best possible combination of traits to improve biomass/yield, we are further dissecting the genetic regulation of resilience using a diverse panel of 200 cowpea accessions. Our findings suggest that breeding for a multi-stage drought-resilient genotype is needed to sustain yield under rainfed conditions
Are we prepared for the new growing challenges ahead?
In addition to drought, another hidden challenge often overlooked is the rising nighttime temperatures, which are increasing at nearly twice the rate of daytime temperatures globally (NOAA, 2023). Compared with drought and daytime heat stress, the effects of warmer nights have been largely understudied in legumes, especially under limited water availability conditions. Warmer nights during critical crop growth stages can increase nocturnal respiration, leading to higher consumption of photosynthates for maintenance instead of growth. This shift reduces carbon allocation to growth and can decrease plant performance and yield in legumes (Sankarapillai et al., 2025).
In response to this critical knowledge gap, our recent research explored how cowpea responds to individual drought or high night temperature and combinations during the reproductive stage (Chakravaram et al., 2025). The findings showed that exposure to each stressor alone resulted in yield losses of less than 30%, and their co-occurrence led to a staggering reduction in yields of more than 50%. This significant drop highlights a concerning synergistic effect where the warmer nights under rainfed conditions can result in greater damage than anticipated.
To address the production and nutrition challenges, breeding programs have been combining classical phenotyping and genomic tools to improve the tolerance of cowpea to drought (Ravelombola et al., 2020, 2018), waterlogging (Basavaraj et al., 2024), heat (Angira et al., 2022), and other stresses (Ravelombola et al., 2017). Recent efforts have utilized the multi-parent advanced generation inter-cross (MAGIC) and other populations to identify genomic regions linked to drought tolerance (Ravelombola et al., 2021), along with desirable agronomic and seed quality traits (Huynh et al., 2018; Angira et al., 2022). These studies highlight the importance of utilizing valuable genetic resources to select superior traits and genotypes to improve food and nutrition security.
Summary
Cowpea is a vital crop for resilient agriculture due to its early maturity, robust nutritional profile, and ability to withstand stress. In the United States, cowpea thrives in a variety of environments, from the hot and dry southern states to the temperate plains of the North, including regions such as Nebraska and Michigan. This ecological adaptability highlights its potential as a viable crop across different agricultural zones. The USDA Germplasm Resources Information Network (USDA-GRIN) maintains a germplasm collection of more than 8,000 cowpea accessions, serving as a valuable resource for exploring genetic variation. This resource can be utilized to identify adaptive traits and genes that enhance yield and nutritional potential under stress. By leveraging cowpea's genetic diversity and natural resilience, further research is needed to develop cultivars that effectively address the challenges of varying growing conditions and food insecurity, ultimately contributing to sustainable agricultural systems for the future.
References
Abebe, B. K., & Alemayehu, M. T. (2022). A review of the nutritional use of cowpea (Vigna unguiculata L. Walp) for human and animal diets. Journal of Agriculture and Food Research, 10, 100383. https://doi.org/10.1016/j.jafr.2022.100383
Angira, B., Zhang, Y., Scheuring, C. F., Zhang, Y., Masor, L., Coleman, J. R., Liu, Y.-H., Singh, B. B., Zhang, H.-B., Hays, D. B., & Zhang, M. (2022). Construction of a single nucleotide polymorphism linkage map and identification of quantitative trait loci controlling heat tolerance in cowpea, Vigna unguiculata (L.) Walp. Molecular Genetics and Genomics, 297(6), 1481–1493. https://doi.org/10.1007/s00438-022-01928-9
Awonaike, K. O., Kumarasinghe, K. S., & Danso, S. K. A. (1990). Nitrogen fixation and yield of cowpea (Vigna unguiculata) as influenced by cultivar and Bradyrhizobium strain. Field Crops Research, 24(3), 163–171. https://doi.org/10.1016/0378-4290(90)90035-A
Basavaraj, P. S., Jangid, K. K., Babar, R., Gangana Gowdra, V. M., Gangurde, A., Shinde, S., Tripathi, K., Patil, D., Boraiah, K. M., Rane, J., Harisha, C. B., Halli, H., Sammi Reddy, K., & Prabhakar, M. (2024). Adventitious root formation confers waterlogging tolerance in cowpea (Vigna unguiculata (L.) Walp.). Frontiers in Sustainable Food Systems, 8. https://doi.org/10.3389/fsufs.2024.1373183
Bista, M. K., & Bheemanahalli, R. (2024). Finger Millet: A climate-resilient and multi-nutrient crop for the uncertain future. CSA News, 69(10), 52–56. https://doi.org/10.1002/csan.21404
Chakravaram, A., Sankarapillai, L. V., Poudel, S., & Bheemanahalli, R. (2025). Interactive effects of drought and high night temperature on physiology and yield components of cowpea (Vigna unguiculata (L.) Walp.). Journal of Agriculture and Food Research, 101844. https://doi.org/10.1016/j.jafr.2025.101844
FAOSTAT. (2023). https://www.fao.org/faostat/en/#data/QCL
Fatokun, C. A., Boukar, O., & Muranaka, S. (2012). Evaluation of cowpea (Vigna unguiculata (L.) Walp.) germplasm lines for tolerance to drought. Plant Genetic Resources, 10(3), 171–176. https://doi.org/10.1017/S1479262112000214
Goufo, P., Moutinho-Pereira, J. M., Jorge, T. F., Correia, C. M., Oliveira, M. R., Rosa, E. A. S., António, C., & Trindade, H. (2017). Cowpea (Vigna unguiculata L. Walp.) Metabolomics: Osmoprotection as a physiological strategy for drought stress resistance and improved yield. Frontiers in Plant Science, 8. https://doi.org/10.3389/fpls.2017.00586
Huynh, B.-L., Ehlers, J. D., Huang, B. E., Muñoz-Amatriaín, M., Lonardi, S., Santos, J. R. P., Ndeve, A., Batieno, B. J., Boukar, O., Cisse, N., Drabo, I., Fatokun, C., Kusi, F., Agyare, R. Y., Guo, Y.-N., Herniter, I., Lo, S., Wanamaker, S. I., Xu, S., … Roberts, P. A. (2018). A multi-parent advanced generation inter-cross (MAGIC) population for genetic analysis and improvement of cowpea (Vigna unguiculata L. Walp.). The Plant Journal: For Cell and Molecular Biology, 93(6), 1129–1142. https://doi.org/10.1111/tpj.13827
Jayathilake, C., Visvanathan, R., Deen, A., Bangamuwage, R., Jayawardana, B. C., Nammi, S., & Liyanage, R. (2018). Cowpea: An overview on its nutritional facts and health benefits. Journal of the Science of Food and Agriculture, 98(13), 4793–4806. https://doi.org/10.1002/jsfa.9074
Lonardi, S., Muñoz-Amatriaín, M., Liang, Q., Shu, S., Wanamaker, S. I., Lo, S., Tanskanen, J., Schulman, A. H., Zhu, T., Luo, M.-C., Alhakami, H., Ounit, R., Hasan, A. Md., Verdier, J., Roberts, P. A., Santos, J. R. P., Ndeve, A., Doležel, J., Vrána, J., … Close, T. J. (2019). The genome of cowpea (Vigna unguiculata [L.] Walp.). The Plant Journal, 98(5), 767–782. https://doi.org/10.1111/tpj.14349
Mallillin, A. C., Trinidad, T. P., Raterta, R., Dagbay, K., & Loyola, A. S. (2008). Dietary fiber and fermentability characteristics of root crops and legumes. British Journal of Nutrition, 100(03), 485–488. https://doi.org/10.1017/S000711450891151X
Matsui, T., & Singh, B. B. (2003). Root characteristics in cowpea related to drought tolerance at the seedling stage. Experimental Agriculture, 39(1), 29–38. https://doi.org/10.1017/S0014479703001108
NOAA. (2023). Annual 2023 Global Climate Report| National Centers for Environmental Information (NCEI). https://www.ncei.noaa.gov/access/monitoring/monthly-report/global/202313
Nunes, C., Moreira, R., Pais, I., Semedo, J., Simões, F., Veloso, M. M., & Scotti-Campos, P. (2022). Cowpea physiological responses to terminal drought-Comparison between four landraces and a commercial variety. Plants, 11(5), Article 5. https://doi.org/10.3390/plants11050593
Owade, J. O., Abong’, G., Okoth, M., & Mwang’ombe, A. W. (2020). A review of the contribution of cowpea leaves to food and nutrition security in East Africa. Food Science & Nutrition, 8(1), 36–47. https://doi.org/10.1002/fsn3.1337
Poudel, S., Valsala Sankarapillai, L., Sivarathri, B. S., Hosahalli, V., Harkess, R. L., & Bheemanahalli, R. (2025). Characterization of cowpea genotypes for traits related to early-season drought tolerance. Agriculture, 15(10), 1075. https://doi.org/10.3390/agriculture15101075
Ravelombola, W., Shi, A., Chen, S., Xiong, H., Yang, Y., Cui, Q., Olaoye, D., & Mou, B. (2020). Evaluation of cowpea for drought tolerance at seedling stage. Euphytica, 216(8), 123. https://doi.org/10.1007/s10681-020-02660-4
Ravelombola, W., Shi, A., & Huynh, B.-L. (2021). Loci discovery, network-guided approach, and genomic prediction for drought tolerance index in a multi-parent advanced generation intercross (MAGIC) cowpea population. Horticulture Research, 8(1), 1–13. https://doi.org/10.1038/s41438-021-00462-w
Ravelombola, W., Shi, A., Qin, J., Weng, Y., Bhattarai, G., Zia, B., Zhou, W., & Mou, B. (2018). Investigation on Various Aboveground Traits to Identify Drought Tolerance in Cowpea Seedlings. HortScience, 53(12), 1757–1765. https://doi.org/10.21273/HORTSCI13278-18
Ravelombola, W. S., Shi, A., Weng, Y., Clark, J., Motes, D., Chen, P., & Srivastava, V. (2017). Evaluation of salt tolerance at germination stage in cowpea [Vigna unguiculata (L.) walp]. HortScience, 52(9), 1168–1176. https://doi.org/10.21273/HORTSCI12195-17
Sankarapillai, L. V., Adhikari, B., Bista, M. K., Shrestha, A., Stetina, S. R., Reddy, K. R., & Bheemanahalli, R. (2025). High night temperature disrupts the assimilate utilization and yield potential in soybean. Plant Stress, 100826. https://doi.org/10.1016/j.stress.2025.100826
Seki, M., Umezawa, T., Urano, K., & Shinozaki, K. (2007). Regulatory metabolic networks in drought stress responses. Current Opinion in Plant Biology, 10(3), 296–302. https://doi.org/10.1016/j.pbi.2007.04.014
Sheahan, C. M. (2012). Plant guide for cowpea (Vigna unguiculata). USDA-Natural Resources Conservation Service, Cape May Plant Materials Center, Cape May, NJ.
Singh, S. K., & Reddy, K. R. (2011). Regulation of photosynthesis, fluorescence, stomatal conductance and water-use efficiency of cowpea (Vigna unguiculata [L.] Walp.) under drought. Journal of Photochemistry and Photobiology B: Biology, 105(1), 40–50. https://doi.org/10.1016/j.jphotobiol.2011.07.001
Singini, M., Chipeta, M. M., Njira, K., & Fiwa, L. (2024). Dissecting cowpea genetic variability under moisture stress conditions and implications for drought tolerance breeding. International Journal of Agronomy, 2024(1), 5358207. https://doi.org/10.1155/2024/5358207
Turk, K. J., Hall, A. E., & Asbell, C. W. (1980). Drought adaptation of cowpea. I. Influence of drought on seed yield. Agronomy Journal, 72(3), 413–420. https://doi.org/10.2134/agronj1980.00021962007200030004x
USDA. (2019). FoodData Central. https://fdc.nal.usda.gov/food-details/175208/nutrients
Members Forum
This article is part of our Members Forum—a place to share your work or opinions and perspectives on any issue relevant to our members. The views and opinions expressed in this column are not necessarily those of the publisher. Do you have a perspective on a particular issue that you’d like to share with fellow members? Submit it to CSA News. Submissions should be 800 words or less and may be subject to review by our editors-in-chief.
Text © . The authors. CC BY-NC-ND 4.0. Except where otherwise noted, images are subject to copyright. Any reuse without express permission from the copyright owner is prohibited.