Fostering Microbial Activity and Diversity in Agricultural Systems
Adopting Better Management Practices and Strategies: Part 2
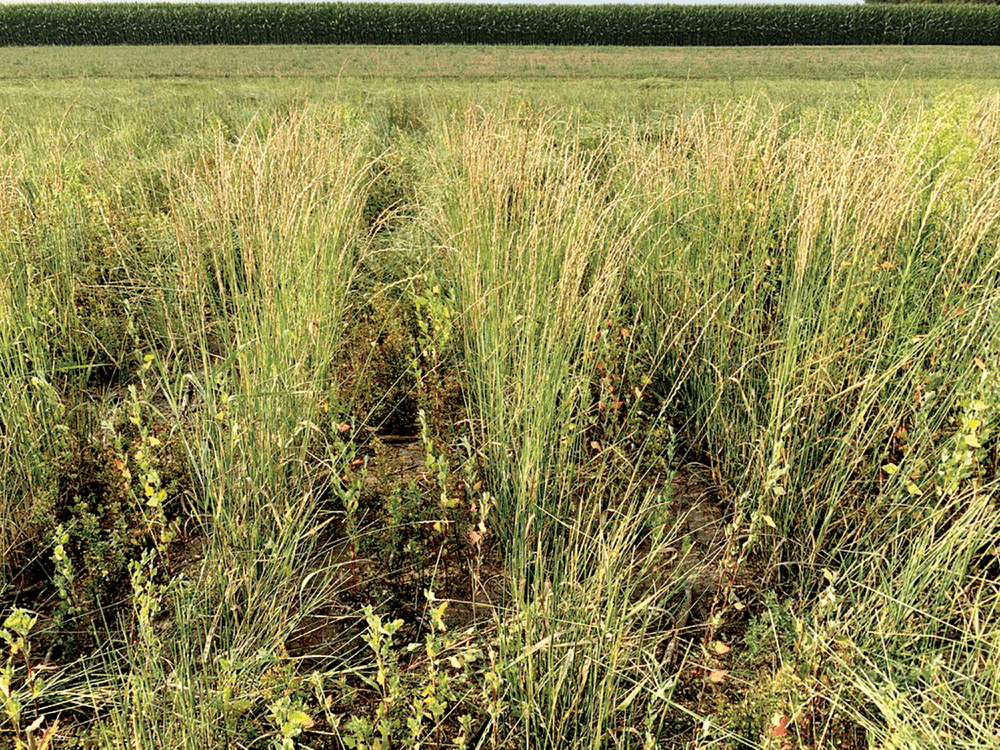
This article is the second in a three-part series on fostering microbial activity and diversity through better management practices and strategies. Part 2 will discuss management practices for better microbial activity and diversity.
Microorganisms play a key role in agriculture and soil health by improving nutrient cycling, organic matter decomposition, water‐holding capacity, soil aeration, pH balance, soil structure, etc. Thus, to maximize the benefits of microbial activity, we need to improve the farming practices to improve their abundance and diversity. There are several agronomic and cultural practices farmers can adopt to foster the microbial communities and their interaction with plants to maximize their crop productivity and, in the long term, improve their soil health and sustainability. Higher microbial diversity is also correlated to the increased functional diversity and resilience against different environmental stresses. Improved management practices create favorable conditions for microbial proliferation, leading to their higher activity and abundance and strengthened resilience and stability against environmental disturbances.
Management Practices for Better Microbial Activity and Diversity
Crop Diversification
Crop diversification encompasses the cultivation of various crop species in a particular farm or field. This can occur simultaneously (intercropping) or in rotation (crop rotation).
Intercropping involves growing different crop species in the same field during a particular season and is also referred to as polyculture or mixed cropping systems. Commonly grown species include cereals, pulses, and other crops. This practice improves soil bulk density, soil aeration, nutrient mobilization, and soil aggregation and harbors different microbes, thus improving microbial activity and overall soil health. For instance, intercropping of corn with faba bean or pigeon pea has been shown to improve soil micro‐ and macro‐aggregation, microbial biomass, and enzymatic activities (Garland et al., 2017; Tian et al., 2019).
Crop rotation involves growing different crops on the same field in a sequenced pattern or cycle. For example, rotating soybean and maize reduces pathogenic microbial communities while enhancing beneficial microbes in the soil (Sun et al., 2023). The interaction of the soil microbiome with different crops improves soil organic carbon and nutrients; also, avoiding the repetition of particular crops breaks the pathogen life cycle, reducing disease pressure. Thus, crop diversification promotes crop productivity through soil health and the soil microbiome.
Cover Crops
Cover crops consist of various crop species grown primarily to enhance soil health. These species include grasses like rye, oat, and barley; legumes like clover, lentil, and vetch; and brassicas like radishes and mustards. Cover crops offer various benefits to soil health, including erosion control, soil fertility improvement, weed suppression, soil moisture maintenance, breaking of compact soil layers, and improving nutrient mobilization (Poeplau & Don, 2015; Blanco‐Canqui et al., 2011). For example, legume cover crops like lentils and vetch are capable of fixing atmospheric nitrogen and releasing abundant amino acids and sugars as root exudates, thereby promoting beneficial bacterial and fungal communities (Cazzaniga et al., 2023). Thapa et al. (2021) observed that incorporating cover crops in a mixture with legumes improved soil fungal abundance, enzymatic activities, and overall soil biological health under wheat–sorghum rotations in semi‐arid ecosystems. Similarly, Chavarria et al. (2016) reported that utilizing a mixture of cover crops as a crop diversification strategy improves soil microbial diversity and enzyme activities in corn–soybean cropping systems under humid conditions. Thus, cover crop adoption promotes a sustainable future for soil health and the soil microbiome.
Mulching
Mulching is a practice of reducing the exposure of the soil surface area to direct sunlight, which checks moisture evaporation and weed growth and maintains moisture and temperature in the soil, promoting soil microbial activity and abundance. Mulching serves as a positive factor for increasing crop growth, yield, and use of water and nutrients (Lal, 1974; Mulumba & Lal, 2008; Qin et al., 2015). The mulching materials can be dead plants and their parts, artificial mulch like plastic, and living mulches like cover crops. Several studies have reported the enrichment of bacterial and fungal activities and abundance due to mulching with more positive results in organic mulching (Tian et al., 2022; Zhang et al., 2020). Thus, mulching can be a very effective tool in boosting crop yield, soil health, and microbial activity and abundance in soil and promoting climate‐resilient agriculture.
Livestock Manure
Raising livestock at farms can be beneficial for meat and milk purposes as well as for manure. Livestock manure has been used as a source of nutrients for centuries in agriculture. Several studies have shown application of dairy manure, poultry manure, and other animal manures increased soil organic matter and organic carbon, nitrogen, and water‐holding capacity in the soil and was associated with higher microbial biomass, activity, and abundance (Rayne & Aula, 2020; Wan et al., 2021). One‐hundred‐year‐old experiments on applying manure in the Knorr‐Holden Plot in Nebraska have shown increased soil organic matter, nitrogen, and phosphorus in the soil and provided stable yield and sustainable maize production systems (Maharjan et al., 2021). Some manures like poultry manure supply more nitrogen than other manures and also differ in other nutrients. Thus, different manures hold different physical and chemical properties, and applying them at different combinations can be beneficial in enhancing microbial activity and their abundance and ultimately enhancing nutrient mobilization and resulting in better soil and higher crop productivity.
Manure itself has various microbial communities and is rich in nutrients. Jangid et al. (2008) observed the addition of poultry litter improved the bacterial richness and evenness. The addition of liquid swine manure reduced the pathogenic microbial communities and pathogen infestation in potato fields (Conn & Lazarovits, 1999). The addition of manure also increased the organic carbon inputs, improving the soil organic carbon (Haynes & Naidu, 1998).
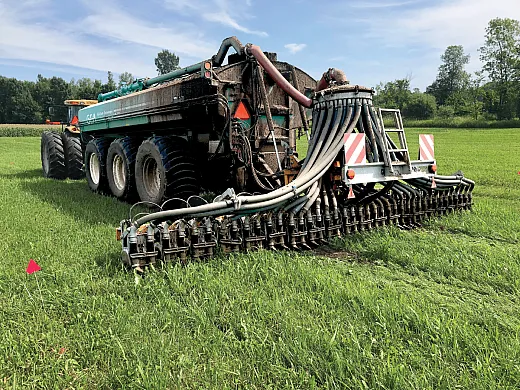
Organic Amendments
Organic amendments are various organic inputs in the soil to improve soil biological activity and overall soil health through microbial activity and abundance. These include crop residue, manure, composts, biochar, and many others. When crop residues are reintegrated into the soil, they serve as a vital organic carbon and nutrient for crops and soil microbes. As these residues decompose, they augment microbial biomass, activity, and diversity, thereby fostering soil aggregation and soil health. Moreover, the ongoing decomposition of these residues contributes to the formation of soil humic mass and recalcitrant organic matter, further enriching soil quality. For instance, maize and sugarcane crop residues enhance the humic mass content and soil aggregation, thereby promoting soil biological health (Liu et al., 2021; Zhang et al., 2021). Biochar, a charcoal‐like substance that is enriched in carbon, is produced by burning organic materials from various agricultural sources. Its intricate structure renders it highly resistant to microbial decomposition, thereby enhancing soil’s capacity to sequester organic matter and nutrients. Additionally, biochar application enhances the soil microbiome, leading to improved wheat performance and mitigated pesticide accumulation in wheat by increasing root–microbiome interaction, particularly under stressful conditions (Meng et al., 2019). Similarly, compost production involves the decompositions of the waste and humus materials, yielding a valuable fertilizer and nutrient source for crop production. Application of compost enhances nutrient availability and improves the growth and productivity of crops such as corn, wheat, etc. (Aiad et al., 2021).
Plant Growth‐Promoting Microorganisms
Introduction of beneficial microorganisms like nitrogen‐fixing bacteria and mycorrhizae through inoculation have been shown to improve crop production and soil health (Li et al., 2022). Microbial inoculation requires using proper methods that consider crop species, soil condition, and the environment for better results (Lopes et al., 2021). Understanding the interaction of plant–microbial association is key to successfully adopting application of microbial inoculum as biofertilizer for enhancing crop production and reducing fertilizer inputs. Abd‐Alla et al. (2014) observed that dual inoculation of rhizobia and arbuscular mycorrhizal fungi in faba beans improved crop performance through efficient acquisition of soil nutrients and nitrogen fixation while studies reported yield increase in corn, soybean, and cotton (Megali et al., 2015; Khaitov et al., 2019). Inoculation of microbial communities, especially the native microbiome, enhances soil microbial diversity, restores the soil health, and increase crop productivity (May et al., 2023; Zhou et al., 2023). Thus, inoculation of microbial communities as biofertilizers holds a strong promise for improving soil health and crop yield through fostering microbial communities.
Reduced Tillage
Tillage exerts disturbance in soil, causing the breakage of soil aggregates and exposing both the soil and its microbiome to sunlight. This alters the physical and chemical properties of the soil, leading to the decomposition of carbon protected within aggregates. Consequently, it results in low organic matter, diminished water and nutrient retention capacity, and a shift in the soil microbiome compositions (Rieke et al., 2022). Reduced‐tillage practices, on the other hand, enhance soil organic matter content, which serves as a vital energy and nutrient source for the microbial community, fostering the increase in microbial activity, altering microbial composition, and promoting the arbuscular mycorrhizal fungi and bacteria involved in decomposing organic matter (Hungria et al., 2009; Van Groenigen et al., 2010). Parajuli et al. (2021) observed that reduced tillage improves soil organic carbon, microbial biomass, and microbial activity in corn–cotton–soybean rotation systems while boosting the active pool of organic carbon and nutrients in the soil. Thus, reduced tillage emerges as an alternative approach to enhance crop productivity and soil health by fostering increased microbial activity and abundance.
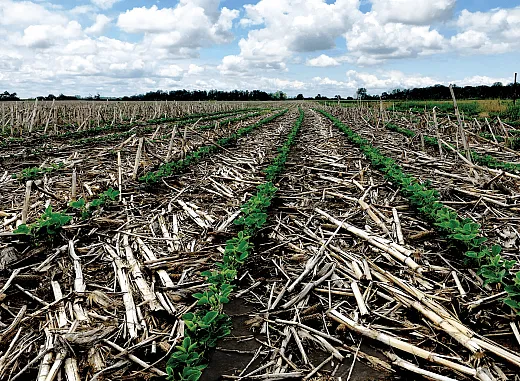
Soil Conservation
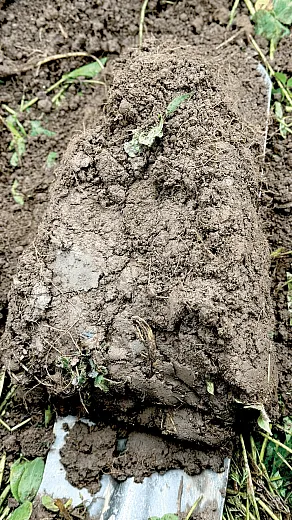
Soil conservation practices like no‐till or reduced tillage, residue retention, cover cropping, diversified and extended crop rotations, and other soil management practices hold a strong promise in promoting soil health by increasing soil organic matter, soil organic carbon and nitrogen, soil aggregates, high microbial activity, and other physical and chemical properties of soil. Soil microbiomes mediate the soil aggregation through cementation and binding of soil particles by releasing different metabolites and biofilms (Kremer & Veum, 2020). Soil aggregates further help in improving soil fertility and crop productivity, along with increasing soil microbial biomass, microbial enzyme activity, and microbial abundance (Veum et al., 2015; Pellegrino et al., 2022), thus reflecting soil conservation practices as a tool in soil health and soil microbiomes.
Precision Agriculture
Precision agriculture holds strong promise for maintaining soil health and microbial activity in soil. With the help of precision agriculture tools, farmers can monitor the plant growth and soil moisture and nutrient status in real time. This helps in optimizing irrigation and nutrient management, and thus, maximizing the microbial activity and abundance in soil, which will ultimately help in mobilizing nutrients, decomposing soil organic matter, and increasing organic carbon and nitrogen content in soil (Borowik & Wyszkowska, 2016). Similarly, precision tools mediate disease, and pest monitoring helps to reduce the application of pesticides in the field, which will again reduce their negative impacts on soil microbiomes (Lo et al., 2010). Thus, precision agriculture opens several avenues in monitoring plant and soil health and different functional properties and traits, thereby, adopting and improving farming practices to improve soil health and soil microbiomes and attain better yields and productivity.
Inorganic Fertilizers
Fertilizers are essential components of agriculture, impacting crop growth, development, and ultimately, yield. The choice of fertilizers and their dosages influences soil microbiomes. The effects of fertilization on soil microbiomes are multifaceted. For instance, a long‐term study spanning 55 years has shown no significant impact of inorganic fertilizers on soil microbial biomass and community composition, whereas short‐term effects have been reported as either positive or negative on yield (Williams et al., 2013; O’Donnell et al., 2001). Thus, proper dosage of inorganic fertilizers improve soil fertility and nutrient availability, thereby improving microbial activity and abundance.
Sanitation
Sanitation is another important step in adopting better farm practices to increase microbial activity and abundance in the soil. Proper disposal and removal of diseased plants and their organs, along with sanitary measures to check the infestation and spread of diseases and pests from neighboring fields, will reduce the disease pressure and thus frequency of pesticide application in the field (Salamanca, 2015). The reduced application of pesticides will benefit beneficial soil microbes and maintain the health of the soil.
Soil Testing
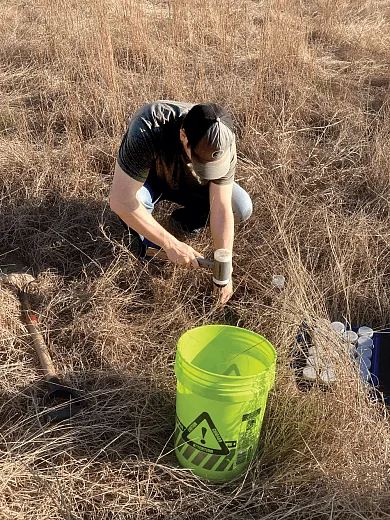
Soil testing in the field can be crucial in decision‐making regarding agricultural practices aimed at maximizing soil health and crop productivity, considering microbial activity, abundance, and compositions (Karlen et al., 2019). Soil tests help understand nutrient content, pH, and electrical conductivity, facilitating the design of fertigation schedules. Measuring bulk density aids in determining the amount of additional manure needed to improve soil aeration. Additionally, DNA sampling from soil helps identify microbial diversity and abundance. Thus, adopting a strategy to increase microbial diversity and abundance will ultimately enhance nutrient recycling and boost crop productivity.
Soil Replacement and Landscape Rehabilitation
Innovative land management strategies, such as topsoil replacement in eroded soils, have been shown to bolster soil microbial activities, enhance soil health, and increase crop yield (Schneider et al., 2021, 2023). Topsoil replacement will be an important strategy in a landscape prone to soil erosion where soil fertility and soil health have been diminished to the extent of making the area unfit for crop cultivations. Schneider et al. (2023) reported that soil landscape rehabilitation increased organic carbon, nutrient availability, water infiltration rate, fungal and bacterial populations, and overall soil health, which indicates that rejuvenating the soil’s microbial activity and abundance will help in rehabilitating the poor soil into cultivable soil.
Soil Fertility and Nutrient Management
The biodiversity of microbial populations is essential in soil fertility and nutrient management. These diverse populations of microbes are critical for the mobilization of nutrients for plant availability, rhizosphere interactions, and many other functions integral to soil and plant health (Sabir et al., 2021). Given the niche role various microbes play in soil and plant health, it’s important to recognize how different nutrient management strategies can affect microbial populations and activities. A beneficial management practice that can bolster microbial communities and soil health is using organic fertilizers in agricultural systems. Most agronomic systems implement inorganic fertilizers to boost crop growth by providing plant‐available nutrients; however, the extensive use of these fertilizers has resulted in acidification of our soils, leading to repercussions on native microbial communities (Ozlu & Kumar, 2018). An alternative to chemical fertilizers has been organic fertilizers, derived from plant or animal‐based materials. These organic fertilizers have the potential to enhance biogeochemical cycling, provide slow‐release plant‐essential nutrients for plant uptake, and increase the diversity of rhizosphere microbial populations (Yu et al., 2024). The significance of the effects on microbial communities and soil health can vary depending on the source of the organic fertilizer; however, organic fertilizer serves as a beneficial alternative that can enrich microbial diversity and activity (Cesarano et al., 2017; Yu et al., 2024).
Irrigation Management
Soil moisture is a critical factor in maintaining microbial diversity, impacting various aspects of microbial life from respiration to metabolic functioning (Borowik & Wyszkowska 2016; Van Horn et al., 2014). Different moisture levels can lead to diverse responses within microbial communities with some species thriving in moist conditions and others preferring slightly drier environments, influenced by acidity and alkalinity levels induced by varying moisture levels. Additionally, moisture content also affects nutrient availability, shaping the composition and distribution of the microbial populations. Soil texture also influences microbial activity under moisture stress as demonstrated by recent research (Siebielec et al., 2020) showing that loamy soil exhibits greater resilience in bacterial activity during drought stress compared with sandy soil. Some studies also reported that increases in rainfall can elevate methane flux and influence microbial biomass and organic carbon contents (Wu et al., 2021). Therefore, effective moisture management, such as through irrigation, is essential for sustaining soil health and microbial communities, ultimately contributing to increased crop productivity.
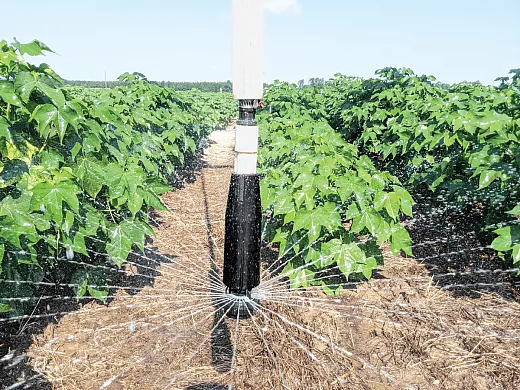
Disease and Pest Management
Another benefit of a diverse microbiome is its contribution to soil–plant system resilience against soil‐borne pathogens and pests. Conventional agriculture has typically used pesticides to reduce the effects of disease and pests on crop health and yields. However, pesticide usage can have ramifications on indigenous soil microbial communities; affecting the microbial diversity and activity in the soil, along with posing the potential risk to environmental and human health (Lo, 2010; Rani et al., 2021). Studies have shown that a diverse, functional microbiome can inhibit the development of diseases by combating soil‐borne pathogens and insect pests (Hu et al., 2016; Wang & Li, 2019; Francis et al., 2020). In the study performed by Hu et al. (2016), it was reported that greater Pseudomonas density and diversity negatively correlated with pathogen density, leading to decreased disease incidence.
Due to the ability of active and diverse microbiomes to suppress disease and pests both directly and indirectly, microbes have been implemented as biocontrol agents as part of an integrated pest management (IPM) strategy and as an alternative to pesticides (Elnahal et al., 2022). The addition of microbial inocula of native soil microbes has been explored as disease and pest control management, manipulating the microbiome to enhance “soil immunity” and protecting crops through plant–microbe relationships (Gadhave et al., 2016). Other management strategies that can assist with disease and pest suppression are those that magnify microbial diversity such as crop rotation (Peralta et al., 2018) and the addition of organic amendments (Akanmu et al., 2021).
References
Abd‐Alla, M. H., El‐Enany, A. W. E., Nafady, N. A., Khalaf, D. M., & Morsy, F. M. (2014). Synergistic interaction of Rhizobium leguminosarum bv. viciae and arbuscular mycorrhizal fungi as a plant growth promoting biofertilizers for faba bean (Vicia faba L.) in alkaline soil. Microbiological research, 169(1), 49–58.
Aiad, M. A., Amer, M. M., Khalifa, T. H., Shabana, M. M., Zoghdan, M. G., Shaker, E. M., … & Kheir, A. M. (2021). Combined application of compost, zeolite and a raised bed planting method alleviate salinity stress and improve cereal crop productivity in arid regions. Agronomy, 11(12), 2495.
Akanmu, A. O., Babalola, O. O., Venturi, V., Ayilara, M. S., Adeleke, B. S., Amoo, A. E., … & Glick, B. R. (2021). Plant disease management: leveraging on the plant‐microbe‐soil interface in the biorational use of organic amendments. Frontiers in Plant Science, 12, 700507.
Blanco‐Canqui, H., Mikha, M. M., Presley, D. R., & Claassen, M. M. (2011). Addition of cover crops enhances no‐till potential for improving soil physical properties. Soil Science Society of America Journal, 75(4), 1471–1482.
Borowik, A., & Wyszkowska, J. (2016). Soil moisture as a factor affecting the microbiological and biochemical activity of soil. Plant, Soil and Environment, 62(6), 250–255.
Cazzaniga, S. G., Braat, L., van den Elsen, S., Lombaers, C., Visser, J., Obinu, L., … & Helder, J. (2023). Pinpointing the distinctive impacts of ten cover crop species on the resident and active fractions of the soil microbiome. Applied Soil Ecology, 190, 105012.
Cesarano, G., De Filippis, F., La Storia, A., Scala, F., & Bonanomi, G. (2017). Organic amendment type and application frequency affect crop yields, soil fertility and microbiome composition. Applied Soil Ecology, 120, 254–264.
Chavarría, D.N., Verdenelli, R.A., Serri, D.L., Restovich, S.B., Andriulo, A.E., Meriles, J.M., & Vargas‐Gil, S. (2016). Effect of cover crops on microbial community structure and related enzyme activities and macronutrient availability. European journal of soil biology, 76, 74–82.
Conn, K. L., & Lazarovits, G. (1999). Impact of animal manures on verticillium wilt, potato scab, and soil microbial populations. Canadian Journal of Plant Pathology, 21(1), 81–92.
Elnahal, A. S., El‐Saadony, M. T., Saad, A. M., Desoky, E. S. M., El‐Tahan, A. M., Rady, M. M., … & El‐Tarabily, K. A. (2022). The use of microbial inoculants for biological control, plant growth promotion, and sustainable agriculture: A review. European Journal of Plant Pathology, 162(4), 759–792.
Francis, F., Jacquemyn, H., Delvigne, F., & Lievens, B. (2020). From diverse origins to specific targets: role of microorganisms in indirect pest biological control. Insects, 11(8), 533.
Gadhave, K. R., Hourston, J. E., & Gange, A. C. (2016). Developing soil microbial inoculants for pest management: can one have too much of a good thing? Journal of chemical ecology, 42, 348–356.
Garland, G., Bünemann, E. K., Oberson, A., Frossard, E., & Six, J. (2017). Plant‐mediated rhizospheric interactions in maize‐pigeon pea intercropping enhance soil aggregation and organic phosphorus storage. Plant and Soil, 415, 37–55.
Haynes, R. J., & Naidu, R. (1998). Influence of lime, fertilizer and manure applications on soil organic matter content and soil physical conditions: a review. Nutrient Cycling in Agroecosystems, 51, 123–137.
Hu, J., Wei, Z., Friman, V. P., Gu, S. H., Wang, X. F., Eisenhauer, N., … & Jousset, A. (2016). Probiotic diversity enhances rhizosphere microbiome function and plant disease suppression. MBio, 7 (6). https://doi.org/10.1128/mbio.01790‐16
Hungria, M., Franchini, J. C., Brandao‐Junior, O., Kaschuk, G., & Souza, R. A. (2009). Soil microbial activity and crop sustainability in a long‐term experiment with three soil‐tillage and two crop‐rotation systems. Applied Soil Ecology, 42(3), 288–296.
Jangid, K., Williams, M. A., Franzluebbers, A. J., Sanderlin, J. S., Reeves, J. H., Jenkins, M. B., … & Whitman, W. B. (2008). Relative impacts of land‐use, management intensity and fertilization upon soil microbial community structure in agricultural systems. Soil Biology and Biochemistry, 40(11), 2843–2853.
Karlen, D. L., Veum, K. S., Sudduth, K. A., Obrycki, J. F., & Nunes, M. R. (2019). Soil health assessment: Past accomplishments, current activities, and future opportunities. Soil and Tillage Research, 195, 104365.
Khaitov, B., Allanov, K., Islam, K. R., & Park, K. W. (2019). Bio‐inoculant improves nitrogen‐use efficiency and cotton yield in saline soils. Journal of Plant Nutrition and Soil Science, 182(3), 393–400.
Kremer, R. J., & Veum, K. S. (2020). Soil biology is enhanced under soil conservation management. In soil and water conservation: A celebration of 75 years (pp. 203–211). Soil and Water Conservation Society.
Lal, R. (1974). Soil temperature, soil moisture and maize yield from mulched and unmulched tropical soils. Plant and Soil, 40, 129–143.
Li, J., Wang, J., Liu, H., Macdonald, C. A., & Singh, B. K. (2022). Application of microbial inoculants significantly enhances crop productivity: a meta‐analysis of studies from 2010 to 2020. Journal of Sustainable Agriculture and Environment, 1(3), 216–225.
Liu, H., Qi, Y., Wang, J., Jiang, Y., & Geng, M. (2021). Synergistic effects of crop residue and microbial inoculant on soil properties and soil disease resistance in a Chinese Mollisol. Scientific Reports, 11(1), 24225.
Lo, C. C. (2010). Effect of pesticides on soil microbial community. Journal of Environmental Science and Health Part B, 45(5), 348–359.
Lopes, M. J. D. S., Dias‐Filho, M. B., & Gurgel, E. S. C. (2021). Successful plant growth‐promoting microbes: inoculation methods and abiotic factors. Frontiers in Sustainable Food Systems, 5, 606454.
Maharjan, B., Das, S., Nielsen, R., & Hergert, G. W. (2021). Maize yields from manure and mineral fertilizers in the 100‐year‐old Knorr–Holden Plot. Agronomy Journal, 113(6), 5383–5397.
May, A., Coelho, L. F., Pedrinho, A., Batista, B. D., Mendes, L. W., Mendes, R., … & Vilela, E. S. D. (2023). The use of indigenous bacterial community as inoculant for plant growth promotion in soybean cultivation. Archives of Agronomy and Soil Science, 69(1), 135–150.
Megali, L., Schlau, B., & Rasmann, S. (2015). Soil microbial inoculation increases corn yield and insect attack. Agronomy for Sustainable Development, 35, 1511–1519.
Meng, L., Sun, T., Li, M., Saleem, M., Zhang, Q., & Wang, C. (2019). Soil‐applied biochar increases microbial diversity and wheat plant performance under herbicide fomesafen stress. Ecotoxicology and Environmental Safety, 171, 75–83.
Mulumba, L. N., & Lal, R. (2008). Mulching effects on selected soil physical properties. Soil and Tillage Research, 98(1), 106–111.
O’donnell, A. G., Seasman, M., Macrae, A., Waite, I., & Davies, J. T. (2001). Plants and fertilisers as drivers of change in microbial community structure and function in soils. Plant and Soil, 232, 135–145. Ozlu, E., & Kumar, S. (2018). Response of soil organic carbon, pH, electrical conductivity, and water stable aggregates to long‐term annual manure and inorganic fertilizer. Soil Science Society of America Journal, 82(5), 1243–1251.
Pellegrino, E., Piazza, G., Helgason, T., & Ercoli, L. (2022). Microbiome structure and interconnection in soil aggregates across conservation and conventional agricultural practices allow to identify main prokaryotic and fungal taxa related to soil functioning. Soil Biology and Biochemistry, 175, 108833.
Peralta, A. L., Sun, Y., McDaniel, M. D., & Lennon, J. T. (2018). Crop rotational diversity increases disease suppressive capacity of soil microbiomes. Ecosphere, 9(5), e02235.
Poeplau, C., & Don, A. (2015). Carbon sequestration in agricultural soils via cultivation of cover crops–A meta‐analysis. Agriculture, Ecosystems & Environment, 200, 33–41.
Qin, W., Hu, C., & Oenema, O. (2015). Soil mulching significantly enhances yields and water and nitrogen use efficiencies of maize and wheat: a meta‐analysis. Scientific Reports, 5(1), 16210.
Rani, L., Thapa, K., Kanojia, N., Sharma, N., Singh, S., Grewal, A. S., … & Kaushal, J. (2021). An extensive review on the consequences of chemical pesticides on human health and environment. Journal of Cleaner Production, 283, 124657.
Rayne, N., & Aula, L. (2020). Livestock manure and the impacts on soil health: A review. Soil Systems, 4(4), 64.
Rieke, E. L., Cappellazzi, S. B., Cope, M., Liptzin, D., Mac Bean, G., Greub, K. L., … & Honeycutt, C. W. (2022). Linking soil microbial community structure to potential carbon mineralization: A continental scale assessment of reduced tillage. Soil Biology and Biochemistry, 168, 108618.
Sabir, M. S., Shahzadi, F., Ali, F., Shakeela, Q., Niaz, Z., & Ahmed, S. (2021). Comparative effect of fertilization practices on soil microbial diversity and activity: an overview. Current Microbiology, 78, 3644–3655.
Salamanca, L. R. (2015). Sanitation is critical to prevent plant diseases. Part 2: Field sanitation. Michigan State University Extension. https://bit.ly/3Va4i9I
Schneider, S. K., Cavers, C. G., Duke, S. E., Schumacher, J. A., Schumacher, T. E., & Lobb, D. A. (2021). Crop responses to topsoil replacement within eroded landscapes. Agronomy Journal, 113(3), 2938–2949.
Schneider, S. K., Sutradhar, A. K., Duke, S. E., Lehman, R. M., Schumacher, T. E., & Lobb, D. A. (2023). Key soil properties and their relationships with crop yields as affected by soil–landscape rehabilitation. Agronomy Journal, 115(5), 2404–2423.
Siebielec, S., Siebielec, G., Klimkowicz‐Pawlas, A., Gałązka, A., Grządziel, J., & Stuczyński, T. (2020). Impact of water stress on microbial community and activity in sandy and loamy soils. Agronomy, 10(9), 1429.
Sun, L., Wang, S., Narsing Rao, M. P., Shi, Y., Lian, Z. H., Jin, P. J., … & Wei, D. (2023). The shift of soil microbial community induced by cropping sequence affect soil properties and crop yield. Frontiers in Microbiology, 14, 1095688.
Thapa, V.R., Ghimire, R., Acosta‐Martínez, V., Marsalis, M.A., & Schipanski, M.E. (2021). Cover crop biomass and species composition affect soil microbial community structure and enzyme activities in semiarid cropping systems. Applied Soil Ecology, 157, 103735.
Tian, L., Yu, S., Zhang, L., Dong, K., & Feng, B. (2022). Mulching practices manipulate the microbial community diversity and network of root‑associated compartments in the Loess Plateau. Soil and Tillage Research, 223, 105476.
Tian, X. L., Wang, C. B., Bao, X. G., Wang, P., Li, X. F., Yang, S. C., … & Li, L. (2019). Crop diversity facilitates soil aggregation in relation to soil microbial community composition driven by intercropping. Plant and Soil, 436, 173–192.
Van Groenigen, K. J., Bloem, J., Bååth, E., Boeckx, P., Rousk, J., Bodé, S., … & Jones, M. B. (2010). Abundance, production and stabilization of microbial biomass under conventional and reduced tillage. Soil Biology and Biochemistry, 42(1), 48–55.
Van Horn, D. J., Okie, J. G., Buelow, H. N., Gooseff, M. N., Barrett, J. E., & Takacs‐Vesbach, C. D. (2014). Soil microbial responses to increased moisture and organic resources along a salinity gradient in a polar desert. Applied and environmental microbiology, 80(10), 3034–3043.
Veum, K. S., Kremer, R. J., Sudduth, K. A., Kitchen, N. R., Lerch, R. N., Baffaut, C., … & Sadler, E. J. (2015). Conservation effects on soil quality indicators in the Missouri Salt River Basin. Journal of Soil and Water Conservation, 70(4), 232–246.
Wan, J., Wang, X., Yang, T., Wei, Z., Banerjee, S., Friman, V. P., … & Shen, Q. (2021). Livestock manure type affects microbial community composition and assembly during composting. Frontiers in Microbiology, 12, 621126.
Wang, L., & Li, X. (2019). Steering soil microbiome to enhance soil system resilience. Critical reviews in microbiology, 45(5‐6), 743–753.
Williams, A., Börjesson, G., & Hedlund, K. (2013). The effects of 55 years of different inorganic fertiliser regimes on soil properties and microbial community composition. Soil Biology and Biochemistry, 67, 41–46.
Wu, J., Wang, H., Li, G., Wu, J., Gong, Y., Wei, X., & Lu, Y. (2021). Responses of CH4 flux and microbial diversity to changes in rainfall amount and frequencies in a wet meadow in the Tibetan Plateau. Catena, 202, 105253.
Yu, Y., Zhang, Q., Kang, J., Xu, N., Zhang, Z., Deng, Y., … & Qian, H. (2024). Effects of organic fertilizers on plant growth and the rhizosphere microbiome. Applied and Environmental Microbiology, 90(2), e01719‐e01723.
Zhang, C., Lin, Z., Que, Y., Fallah, N., Tayyab, M., Li, S., … & Zhang, H. (2021). Straw retention efficiently improves fungal communities and functions in the fallow ecosystem. BMC Microbiology, 21(1), 52.
Zhang, S., Wang, Y., Sun, L., Qiu, C., Ding, Y., Gu, H., … & Ding, Z. (2020). Organic mulching positively regulates the soil microbial communities and ecosystem functions in tea plantation. BMC Microbiology, 20(1), 103.
Zhou, Y., Yang, Z., Liu, J., Li, X., Wang, X., Dai, C., … & Li, X. (2023). Crop rotation and native microbiome inoculation restore soil capacity to suppress a root disease. Nature Communications, 14(1), 8126.
Text © . The authors. CC BY-NC-ND 4.0. Except where otherwise noted, images are subject to copyright. Any reuse without express permission from the copyright owner is prohibited.